Introduction
Materials and Methods
1. Plant materials and growing conditions
2. Light treatment
3. Irrigation water and nutrient solution
4. Growth measurement
5. Photosynthesis measurements
6. Chlorophyll fluorescence measurements
7. Statistical analysis
Results and Discussions
Conclusions
Introduction
The cucumber (Cucumis sativus) is a worldwide cultivated economically important vegetable that is used for fresh consumption, salads and pickles. In Korea, the domestic cucumber cultivation area in 2022 was 3,836 ha, whereas the greenhouse cultivation area was 3,063 ha, accounting for about 80% of the total cultivated area (KOSIS, 2024). In 2020, the hydroponic cultivation area was 30 ha. So, cucumber cultivation in the greenhouse is mainly grown in soil. Farmers required high-quality and vigorous plants for high yields. Furthermore, plant’s quality is influenced by growing conditions. For this reason, delicate cultivation management is required to control the growth of plants. Morphological features, chlorophyll concentration, photosynthetic activities, transpiration rate etc. are some parameters that are useful to understand the proper condition of a plant (Hussain et al., 2019; Morales et al., 2020).
Light is an essential energy source for plant growth. The photosynthesis process and morpho-physiological development of a plant greatly depend on the daily light integral (Choi et al., 2022; Slattery et al., 2017). DLI describes the number of photosynthetic photons accumulated by a plant surface in a square meter over 24 hours (Wang et al., 2021). It is applicable to predict yield and set management strategies for growers. Suitable DLI is created by extending the photoperiod or PPFD (Photosynthetic Photon Flux Density) or their combinations. Nowadays, chlorophyll fluorescence is a quick and non-destructive method for understanding the physiological activities of a plant (Di Mola et al., 2021). It is also a helpful method to identify the DLI effect on plants (Cui et al., 2021). Notably, to ensure cost-effective crop cultivation, proper light management is necessary. Research on different vegetable crops showed that LED light is a useful tool for maintaining proper DLI during crop cultivation in a greenhouse or indoors (Bian et al., 2017; Gao et al., 2020; Mao et al., 2019).
In greenhouse cultivation, irrigation amount is almost entirely dependent on its irrigation system and its application. For this reason, planned and reasonable irrigation is required for cost-effective crop production. Generally, irrigation was done before crops faced moisture stress due to lack of moisture or water content in the soil or substrates. In the soil cultivation system, it is required to determine the irrigation time based on soil moisture considering the type of crops, growth stage, cultivation time, and environment. Compared to hydroponic cultivation, soil-cultivated plants have a large root system. As well as, due to the properties (physical, chemical, and biological) soil has the characteristic of having a high buffering capacity when growing crops, but making it difficult to control water intake.
The leaves and fruits of vegetables contain more than 90% water, and in particular, the water content of cucumbers is 96%, which is the highest among vegetables (Moon and Yu, 2013). Water content in cucumber highly depends on moisture content in the soil of its plant’s root zone. For this reason, timely and appropriate irrigation is an important factor for cucumber cultivation in the greenhouse. An et al. (2021a) reported that the starting point of irrigation in soil-cultivated cucumber plants affected the growth of cucumber and water use efficiency. In addition, the soil temperature decreased after irrigation at high light intensity (light intensity 820 W m-2 at 1 p.m.) and was no effect on sap flow and leaf temperature (An et al. 2021b). There have considerable research done on cucumber plants using tensiometer for greenhouse or open field cultivation but inadequate for controlled environments including light intensity. Therefore, this study aimed to investigate the effect of daily light integral on the growth and physiological response of cucumber plants, total irrigation amount and soil water tension in pot soil culture using a tensiometer.
Materials and Methods
1. Plant materials and growing conditions
This experiment was carried out in a controlled environment condition (320 cm × 370 cm) at the Department of Smart Farm and Agricultural Industry, Kangwon National University, Chuncheon, South Korea. The seeds of cucumber (cv. Asia unchun, Asian seed Co., Seoul, Korea) were sown in a plug tray filled with commercial growing media. After one month, uniform-sized seedlings (30 cm in height with 4 leaves) were transplanted into plastic pots (15 L) containing sandy soil. The room temperature was 25 ± 3℃.
2. Light treatment
Plants were grown under three different light intensities 50 µmol m2 s-1 (LS), 200 µmol m2 s-1 (LM) and 350 µmol m2 s-1 (LH) for different day light integrals (DLI) at 2.88, 11.52 and 20.16 mol m-2 d-1 respectively (Table 1). White color (bar type) LED light was used (ZVAS, Sunghyun Hightech Co. Ltd., Hwaseong, Korea) and the light/dark cycle was set for 16h/8h. A total number of six lights were used for each treatment and these were fixed at 100 cm above from the ground level of the plants. Spectral distributions of this light are 51.7% green, 24.6% blue and 21.4% red.
Table 1.
Photosynthetic photon flux density, photoperiod and daily light integral (DLI) for cucumber plants grown in pot culture for three weeks.
Treatment |
Photosynthetic Photon Flux Density (µmol m2 s-1) |
Photoperiod (h d-1) |
Daily Light Integral (mol m-2 d-1) |
LS | 50 | 16 | 2.88y |
LM | 200 | 11.52 | |
LH | 350 | 20.16 |
3. Irrigation water and nutrient solution
Irrigation was done by tap water using a watering can based on plant requirements (growth appearance and soil condition in the pot). A tensiometer (METER Group AG, Munich, Germany) was used for observing soil water tension (kPa) and irrigation management. Additionally, nutrient solution was provided (100 mL/ plant) in all treatments at 3 to 7 days intervals. Solution contained, NO3-N, NH4-N, P, K, Ca, Mg and SO4-S were added 12, 0.7, 2, 7, 5, 2 and 2 me/L respectively.
4. Growth measurement
Growth parameters of cucumber plants were measured at three weeks after treatment. Plants were uprooted from the soil and their roots were carefully washed with tap water. Plant height, leaf length, and leaf width were measured using a ruler. The leaf area of a specific leaf (10th leaf from the tip of plant) of a plant was estimated by a leaf area meter (LI-3100, Li-Cor Inc., Nebraska, USA). The number of nodes of a plant was counted manually. An electronic balance (PAG4102C, Ohaus Corporation, NJ, USA) was used for checking to shoot and root fresh weights as well as their dry weights. Dry weights were measured after drying them in an oven (JEIO TECH OF-22GW, Daejeon, Korea) for 48 h at 80°C. A chlorophyll meter (SPAD 502, Konica Minolta, Tokyo, Japan) was used for the estimation of chlorophyll concentrations in the leaves of a plant. For SPAD reading, the upper leaf blades of three healthy and fully opened leaves were chosen.
5. Photosynthesis measurements
Photosynthesis rate, transpiration rate, stomatal conductance and intercellular CO2 concentration were estimated at three weeks after treatment in fully expanded leaves using an easily carried photosynthesis meter (LI-6400XT, LI-COR Inc., Lincoln, NE, USA). This survey was conducted under specific conditions (temperature 25°C, airflow 500 µmols-1, CO2 400 µmol mol-1 s-1 and light was based on the treatment) in a leaf chamber (3cm × 2cm).
6. Chlorophyll fluorescence measurements
Chlorophyll fluorescence measurements were done in fully expanded leaves by a portable fluorometer (PAR-FluorPen FP 110/D, Photon Systems Instruments, Drásov, Czech Republic). In this process, initially, leaves were adapted for 30 min using fluorometer leaf clips. After that, fluorescence was inspected by the internal light (LED blue, 470 nm, saturating pulse 2400 µmol photon m-2 s-1) and recorded based on the OJIP protocol of the fluorometer (Kim et al., 2022). Values of chlorophyll fluorescence are presented in arbitrary units (a.u.).
7. Statistical analysis
The experiment was conducted with a completely randomized block design. Significant differences were calculated using IBM SPSS Statistics (version 24 for windows, IBM Corp., Armonk, NY, USA) and analysis of variance (ANOVA) followed by Duncan’s Multiple Range Test (DMRT) at p ≤ 0.05. OriginLab 10.0 software 176 (OriginLab, Northampton, MA, USA) was used for principal component analysis (PCA).
Results and Discussions
At three weeks after treatment, plant height was 25% and 21% significantly higher in LM and LS-treated plants respectively compared to those grown in LH treatment (Table 2). Previous research showed that shaded conditions or low light integral enhanced the growth enlargement of plants. Hamedalla (2022) and Zhang et al. (2020) showed that the plant height of cucumber plants was affected by different light integrals. Other family plants also showed that their height was enlarged by the low light integral in the greenhouse, indoor, and growth chamber (Shawon et al., 2021). Researchers noted that, under low light integral, plants try to reach their nearest source of light energy by enhancing their vertical growth. In this process, plants regulate their carbon assimilation in the cell component of shoot (King, 1990; Wu and Tan, 2002).
Table 2.
Effect of daily light integral (DLI) on cucumber plants grown in pot culture at three weeks after in different light treatments. LS, LM and LH indicate plants were treated by daily light integral at 2.88, 11.52 and 20.16 mol m-2 d-1 respectively.
Treatment | Plant growth parameters per plant | |||||||
Plant height (cm) | Leaf |
Number of nodes (ea) |
Stem diameter (mm) | Shoot | ||||
Length (cm) |
Width (cm) |
Area (cm2) |
Fresh weight (g) |
Dry weight (g) | ||||
LS | 70.38 az | 12.00 b | 16.95 b | 210.00 b | 18.80 a | 5.00 a | 57.60 c | 4.20 c |
LM | 73.03 a | 14.40 a | 20.48 a | 294.60 a | 18.00 a | 5.50 a | 87.40 a | 9.40 a |
LH | 58.23 b | 11.10 c | 15.63 b | 196.33 b | 18.00 a | 5.70 a | 73.40 b | 7.60 b |
The leaf length and leaf width of LM-treated plants were significantly around 20% and 30% higher than those grown in LS and LH treatments respectively (Table 2). However, there was no significant variation between LS and LH-treated plants. Furthermore, the leaf area of a selected leaf of a plant was significantly highest in LM-treated plants among the treatments. It was 40% and 50% higher than those plants were grown in LS and LH treatments consecutively. There was no statistical difference between the leaf area of LS and LH-treated plants. Maybe DLI affected leaf growth in plants of LS treatment. Because of low DLI, plants did not reach enough energy for their physiological activities and development.
The stem diameter and number of nodes act as indicators of the survival abilities of a plant (Grossnickle, 2012). Researchers showed that the survival ability of a plant is better which has a bigger stem compared to those with small stem diameters (Morrissey et al., 2010; Rose and Ketchum, 2003). In this experiment, the number of nodes and stem diameter of the cucumber plants were not significantly different among the treatments (Table 2). Maybe a short-term treatment period is the factor for not statistical difference of these parameters between the treatments. In LM-treated plants, shoot fresh weight was 19% and shoot dry weight was 24% significantly higher compared to LH-treated plants (Table 2). However, shoot fresh weight and shoot dry weight of LS-treated plants were 34% and 55% lower respectively than those plants grown in treatment LH. Although leaf width and leaf area were not significantly different between LS and LH-treated plants, but their cumulative effect impact on shoot fresh weight and shoot dry weight (Table 2).
Durand et al. (2018) showed nutrient uptake of plants and their carbohydrate accumulation in leaves greatly depends on their feature of roots. Root also regulates the source-to-sink ratio of a plant. From Fig. 1 it was observed that, among the treatments root surface, root fresh weight and root dry weight of cucumber plants were significantly lowest in LS-treated plants. These are around 50% lower than those grown in LM treatment.
Low DLI causes less physiological activities of LS-treated plants. For this reason, LS-treated plants required less water which leads to less root growth compared to LM-treated plants (Fig. 1). Gelderen et al. (2018) reported that root growth was influenced by received light conditions (intensity, color and duration) of plants through their effect on root-environment interaction and nutrient uptake. In addition, the well-developed shoot and roots of a plant also indicate their convenient photosynthesis by receiving and utilizing proper light wavelengths (Yousef et al., 2021). However, root surface area, root fresh weight and root dry weight of cucumber plants were not significantly affected between LM and LH-treated plants (Fig. 1).
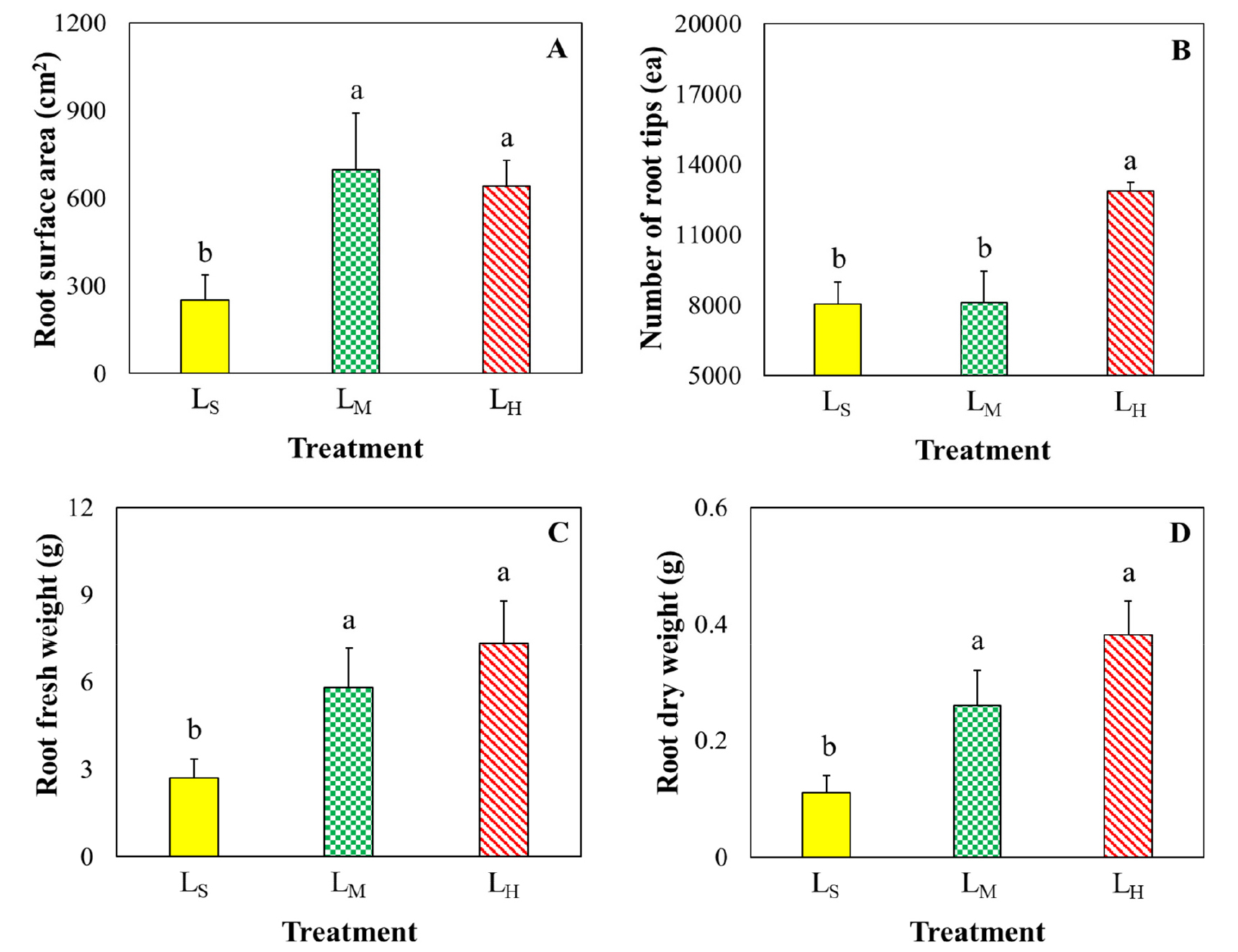
Fig. 1.
Effect of daily light integral on the root surface area (A), number of root tips (B), root fresh weight (C), root dry weight (D) of cucumber plants grown in pot culture at three weeks after treatment. LS, LM and LH indicate plants were treated by daily light integral at 2.88, 11.52 and 20.16 mol m-2 d-1 respectively. The lines above the bar represent the standard deviation of the mean (n = 5). Different letters above the mean bars indicate a significant difference between the treatments by Duncan’s multiple range test (DMRT).
SPAD value is useful to estimation of predicted yield through the relation of dry and fresh weights (Szulc et al., 2021). Table 3 showed that the SPAD value was the lowest (4.41) in LS-treated plants. LM-treated plants showed 16% higher SPAD value than those plants were grown in treatment LS. It indicates growth condition of LS-treated plants was lower than others. Cho et al. (2007) noted that the SPAD value represents the nutritional conditions of plants. Furthermore, there was no significant variation observed in the SPAD value of LM and LH-treated plants (Table 4). Table 2 also showed, shoot dry weight and shoot fresh weight of cucumber plants are higher in LM-treated plants. It make coherent of findings. Ciganda et al. (2012) reported that daily light integral plays a crucial role in the presence of chlorophyll content in a leaf.
Table 3.
Effect of daily light integral on SPAD, photosynthesis rate, stomatal conductance, intercellular CO2 concentration, transpiration rate of cucumber plants grown in pot culture at three weeks after treatment. LS, LM and LH indicate plants were treated by daily light integral at 2.88, 11.52 and 20.16 mol m-2 d-1 respectively.
Treatment |
SPAD (value) |
Photosynthesis rate (µmol CO2 m-2 s-1) |
Stomatal conductance (µmol H2O m-2 s-1) |
Intercellular CO2 concentration (µmol CO2 mol-1) |
Transpiration rate (mol H2O m-2 s-1) |
LS | 41.78 bz | 0.92 b | 0.10 a | 361.23 a | 2.64 a |
LM | 48.48 a | 4.34 a | 0.13 a | 305.01 b | 2.58 a |
LH | 49.33 a | 4.81 a | 0.11 a | 281.99 b | 2.42 a |
Table 4.
Effect of daily light integral on the chlorophyll fluorescence of cucumber plants grown in pot culture at three weeks after treatment. LS, LM and LH indicate plants were treated by daily light integral at 2.88, 11.52 and 20.16 mol m-2 d-1 respectively.
Treatment | Chlorophyll fluorescence | |||||
Fv/Fm | ABS/RC | TRo/RC | ETo/RC | DIo/RC | PIABS | |
LS | 0.84 ay | 1.76 a | 1.47 a | 1.00 a | 0.29 a | 6.51 b |
LM | 0.83 a | 1.73 b | 1.44 b | 1.00 a | 0.29 a | 6.50 b |
LH | 0.82 a | 1.66 c | 1.36 c | 1.00 a | 0.29 a | 7.77 a |
Stomatal conductance was not significantly different between the treatments (Table 3). Maybe the 3-week treatment period and initial stage of plant and the difference in DLI between the treatments are not enough to affects the statistically significant variation in stomatal conductance of cucumber plants. Furthermore, among the treatments, the transpiration rate was also not significantly different (Table 3). Maybe the non-significant variation of stomatal conductance of the plants in different treatments is the reason for the non-significant transpiration rate in the same plants in this study. Prado et al. (2018) noted that the transpiration rate of plants is influenced by their stomatal conductance.
Daily light integral plays a vital role in the photosynthesis activity of a plant (Martín et al., 2015). From Table 3, observed that the photosynthesis rate was around 80% lower in LS-treated plants compared to those were grown under higher light intensities in treatments LM and LH. A similar result was observed in early stage of apple plants, where plants were grown using white LED light and their photosynthesis rate was 50% reduced by low light integral (Choi et al., 2022). Moreover, comparatively reduced leaf and root growth are the reasons for low photosynthetic rate in LS-treated plants (Tables 2, 3 and Fig. 1). Weston et al. (2000) reported that the light acclimation of a plant for photosynthetic purposes greatly depends on its leaf morphology.
For photosynthesis, the intercellular CO2 concentration (Ci) of leaves is an eminent criterion (Tominaga et al., 2018). In this study, Ci was higher in LS-treated plants than in others (Table 3). It is another reason for the lower photosynthesis rate of LS-treated plants. Taylor and Terry (1984) noted that the photochemical energy supply is hindered by the presence of excess CO2 gas in leaves. Zavala et al. (2001) showed that low light integral affected gas exchange resulted inhibits plant growth. However, there was no significant variation between LM and LH-treated plants (Table 3).
The Fv/Fm (maximum quantum yield of photosystem-II) ratios were indicators of photochemical activities and dark-adapted state efficiency in photosystem-II (PSII). In this experiment, significant variation was not observed between the treatments (Table 4). At three weeks after treatment, ABS/RC (absorption per active reaction center) was highest in LS-treated plants and lowest in LH-treated plants. Same as TRo/RC (trapped energy flux per active reaction center) exhibits the highest value in LS-treated plants and the lowest value in LH-treated plants. The driving forces of the ABS/RC and TRo/RC values are relevant to dark reaction of PS-II and generally showed similar trend (Appenroth et al., 2001). As well as, these are affected by different factors including plant age (Krupa and Moniak, 1998). Another research on paprika plants showed that daily light integral affected ABS/RC and TRo/RC values and their response followed a similar trend in plants in different shade conditions (Kim et al., 2022). May be the light integral and age of the plant cumulatively affected ABS/RC and TRo/RC values in different treated plants in this experiment (Table 4). However, the PIABS (performance index on absorption basis) was highest in LH-treated plants. Viljevac et al. (2013) noted that PIABS and ABS/RC showed has inverse relation. This result also supports our findings (Table 4). Furthermore, the ETo/RC (electron transport flux per active reaction center) and DIo/RC (rate of energy dissipated by PSII per reaction center) of cucumber plants were not significantly affected by the different light treatments (Table 4). Maybe a short- term treatment period (three weeks) is not enough for showed statistical differences in Fv/Fm, ETo/RC and DIo/ RC values between the treatments.
At three weeks after treatment, total irrigation was 2.2 L/plant, 5.1 L/plant and 10.2 L/plant in LS, LM and LH treatments (Table 5). The higher daily light integral in LH treatment may lead high evapotranspiration. Resulted, LH-treated plants required more water compared to other treatments, and after the experiment period their cumulative effect showed a significant variation in total irrigation amount in LH treatment than others. It was 5 times and 2 times high as than total irrigation in LS and LM treatments respectively.
Furthermore, soil water tension (SWT) reflects the soil moisture status. SWT was similar in the LS and LM treatments (Fig. 2). Both remained in below -7 kPa and -5kPa respectively. It indicates the presence of water in the soil of these two treatments is enough for plant growth. Continuous supply of water and nutrient solution at regular intervals is the matter of this condition in LS and LM treatments. In addition, treatment LH showed more fluctuations and low values of SWT (up to -30 kPa) compared to the LS and LM treatments. It indicates that among the treatments, comparatively less water content in the soil of LH treatment. High daily light integral (20.16 mol m-2 d-1) causes higher evapotranspiration and leads to presence of less water in the LH treatment than others. Resulted root growth of LH-treated plants was affected (Fig. 1). Although DLI was higher in LH treatment but its low SWT is the reason for similar root growth with LM treated plants. Because SWT indicates the presence of soil water in LH treatment is lower than others. Root growth of plants in the soil is limited by the properties, weather and water conditions of the soil. Less water content in the soil makes it hard and hinders for root penetration. Bengough et al. (2006) noted, that root elongation of plants declined when water channels of soil had mechanical impedance for roots to exploit. Even, root growth may become too clustered to pass through the hard soil resulted, restricting water and nutrient uptake (Passioura, 1991). However, researchers noted that the wilting point of the plant started from -100 kPa of SWT (Shock et al., 2007). In this experiment cucumber plant did not face water scarcity in any treatment.
Relative humidity was around 70% in LM treatment and around 50% in both LS and LH treatments (Table 5). Because of less DLI in LS-treated plants may did not take proper energy for enough photosynthesis which also leads less evapotranspiration and less humidity in LS treatment. On the other hand, DLI was highest in LH treatment but SWT graph indicates the amount of water in pot soil is limited. Plants were grown in pots, for this reason, irrigated water may be evaporated more quickly in LH treatment than others due to high light intensity. Cumulative effect of high light integral and less water in root zone resulted less amount of relative humidity in LH treatment also. Less SWT and moderate DLI may be the reason for 70% RH in LM treatment.
Table 5.
Effect of daily light integral on the total irrigation volume and relative humidity at three weeks after treatment. LS, LM and LH indicate plants were treated by daily light integral at 2.88, 11.52 and 20.16 mol m-2 d-1 respectively.
Treatment |
Total irrigation (L/plant) |
Relative humidity (%) |
LS | 2.2 | 50 ± 5 |
LM | 5.1 | 70 ± 5 |
LH | 10.2 | 50 ± 5 |
The principal component analysis (PCA), represents the correlation between different growth parameters of cucumber plants under different daily light integral (Fig. 3). The lines starting from the middle point of the biplots showed the positive or negative associations of the different growth parameters and their degree of correlation with specific light treatment. It illustrates, 66.25% and 33.75% variabilities in PC1 and PC2 respectively. In addition, two distinct clusters of parameter relation were recognized from this PCA biplot. The graph indicates that the leaf length, leaf width, leaf area, shoot fresh weight, SPAD value, photosynthesis rate and stomatal conductance were positively correlated. In addition, these variables are closer to the LM treatment. From another cluster, plant height, the number of nodes, transpiration rate and intercellular CO2 concentration have a positive correlation, as well as, they are closer to the LS treatment (Fig. 3). Research on different plants also represents a positive correlation between leaf growth and shoot fresh weight of those plants (Kim et al., 2022; Shawon et al., 2023). Choi et al. (2022) also observed that SPAD value and photosynthesis rate in apple seedlings have a positive relation under different intensities of white LED light.
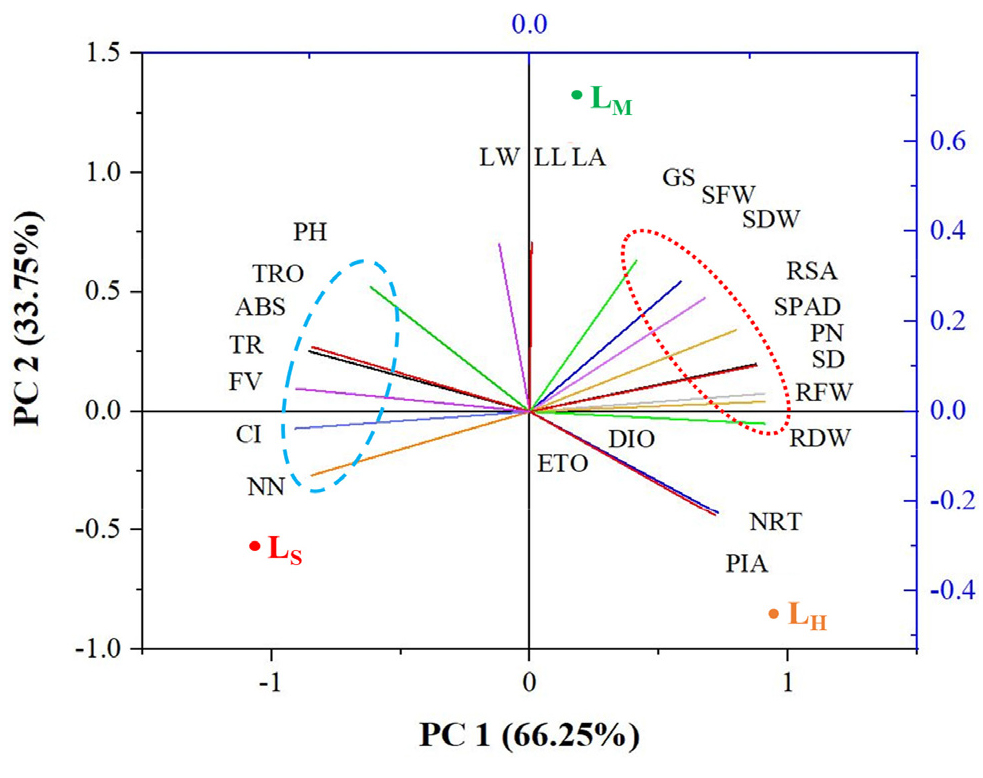
Fig. 3.
Principal component analysis (PCA) interprets relationships among the three treatments and their variables (growth parameters) of cucumber plants grown in pot culture at three weeks after treatment. LS, LM and LH indicate plants were treated by daily light integral at 2.88, 11.52 and 20.16 mol m-2 d-1 respectively. PH-shoot height; LL-leaf length; LW-leaf width; LA-leaf area; NN-number of nodes; SD-stem diameter; RL-root length; SD-stem diameter; SFW- shoot fresh weight; SDW-shoot dry weight; RSA-root surface area; NRT-number of root tips; RFW-root fresh weight; RFW-root dry weight; SPAD-SPAD value; FV-FV/FM; ABS-ABS/RC; TRO-TRo/RC; ETO-ETo/RC; DIO-DIo/RC; PIA-PIABS; PN-Photosynthesis rate; GS-stomatal conductance; CI- Intercellular CO2 concentration and TR-transpiration rate.
Conclusions
This study indicates that daily light integral greatly influenced cucumber plant growth through affected physiological responses. Low DLI 2.88 mol m-2 d-1 is not enough for plant development. DLI 11.52 mol m-2 d-1 is preferable for the growth of cucumber plants in pot culture. Although high DLI 20.16 mol m-2 d-1 showed similar results with LM-treated plants in some parameters including root surface area, root fresh weight and photosynthesis rate but not cost-effective based on irrigation amount. High DLI in LH treatment, decreased soil water tension and required more water. It required approximately double amount of water for irrigation compared to LM treatment. To ensure better yield and quality production of cucumber, further research is needed based on different colors of lights along with their different DLI. Proper irrigation combination is also necessary.