서 론
오이는 시설 내 주년생산으로 꾸준히 재배되며 일본으로 수출량이 2000년에는 5,800톤까지 증가한 중요 작물이고(RDA, 2021), 토마토 또한 건강식품으로 수요가 증가하면서 전국적으로 재배지가 확산된 국내 대표 과채류 중 하나이다(RDA, 2020). 오이는 과채류 중에서도 재배기간이 비교적 짧고 온도, 광, 습도 등의 환경조건에 민감하다(RDA, 2021). 토마토는 모종의 품질이 정식 후 생육과 과실의 품질과 수량에 많은 영향을 미치는 작물이기 때문에 오이와 토마토의 안정적 생산을 위한 고품질 묘 생산 연구는 중요하게 여겨진다(RDA, 2020).
국내 대다수 공정 육묘는 자연광을 이용하는 유리온실 또는 플라스틱온실에서 이루어지며, 이러한 종류의 온실은 광, CO2, 온도, 그리고 습도 등과 같은 외부 환경에 노출되어 매년 일정한 품질의 묘를 생산하기 어렵다(Um 등, 2009). 또한 기후변동성으로 인한 풍수해, 고온해 및 냉해 등의 기상재해 증가로 인하여 균일한 고품질 규격묘 생산이 더욱 어려워질 것으로 예상된다(RDA, 2018). 따라서 재배환경을 인위적으로 조절하여 안정적인 식물생산이 가능하며, 고품질의 작물을 균일하게 생산할 수 있고, 재배기간 단축, 그리고 토지 면적 대비 생산량 증대 등이 장점인 밀폐형 식물생산 시스템(closed-type plant production system, CPPS)을 이용한 육묘 방법이 주목받고 있다(Kozai 등, 2019).
CPPS 내에서 CO2와 광도의 조절이 오이와 토마토 묘 품질에 큰 영향을 미친다는 사실은 널리 알려져 있다. Ahn 등(2003)은 CO2 증가가 토마토 묘의 광합성 효율과 생체중, 건물중 등의 묘소질을 증가시킨다고 보고하였고, Kwack 등(2014)은 광량의 증가가 오이 접목묘의 경경, 건물중 증가에 영향을 미쳤다고 보고하였다. 그리고 Park 등(2020)은 광도와 트레이 규격이 오이와 토마토 묘의 증발산량, 엽수, 생체중 등에 영향을 끼친다고 보고하였다. 하지만 이러한 CPPS 환경조절을 통한 묘소질 향상에 관한 대부분의 연구는 묘의 지상부 생육에 치중되어 있고 묘 지하부 생육에 관한 연구는 부족한 상황이다.
토양 내 무기이온을 흡수하는 주기관인 뿌리의 발달은 양분의 흡수와 토양 미생물과의 상호작용에 영향을 주기 때문에 근권 표면적과 근단 수와 같은 뿌리의 형태가 우수한 묘는 정식 후 뿌리 활착과 양분 흡수에 유리하다(Atwater 등, 2015; Busso 등, 2001; Choi와 Cho, 2019; Foxx와 Kramer, 2020; Hetrick, 1991). 최근 육묘장에서 많이 생산하는 플러그 묘는 뿌리 발달이 어려운 소량의 배지가 담긴 개개의 셀에서 육묘되기 때문에(Jeong 등, 2016), 정식 후 생육을 고려하여 CPPS 내에서 뿌리 발달이 우수한 묘를 생산하기 위한 적정 환경조건을 구명할 필요가 있다. 따라서 본 연구는 CPPS에서 CO2와 광도에 따른 오이와 토마토 묘의 지상부와 지하부 생육을 확인하기 위하여 수행됐다.
재료 및 방법
1. 실험재료 및 재배환경
오이(Cucumis sativus L. ‘Joeunbaekdadagi’)와 토마토(Solanum lycopersicum L. ‘Dotaerang Dia’)를 50구 트레이에 파종하여 밀폐형 식물생산 시스템(C1200H3, FC Poibe Co., Ltd., Seoul, Korea)에서 온도 25±1℃, 상대습도 50±10%의 암 환경에서 4일간 발아시켰다. 발아 후 CO2 농도는 챔버 내의 평균 농도인 500µmol·mol-1(Ambient)을 기준으로 하여, 1,000 그리고 1,500µmol·mol-1로 설정하였다(Fig. 1). 광도는 CPPS에서 경제적으로 이용되는 광도 범위를 기준(Kozai 등, 2019)으로 100, 200 그리고 300±10µmol·m-2·s-1 photosynthetic photon flux density(PPFD)로 설정하였다. 광주기는 12/12 시간(명기/암기)으로 설정하여 22일간 육묘하였다. 광질은 R:B=5:5 LED(ES LEDs Co., Ltd., Seoul, Korea)를 이용하였다(Fig. 2). 육묘 기간 양수분 관리는 온실 다용도 액비를 사용하여(Table 1), 2일 간격으로 저면관수하였다. 실험 동안 밀폐형 식물생산 시스템의 CO2 농도는 데이터 로거(TR-76Ui, T&D Co., Ltd., Matsumoto, Japan)를 이용하여 수집하였다.
Table 1.
Composition of the nutrient solution used in the experiment.
2. 생육 조사
처리 후 22일 차에 초장, 경경, 엽수, 엽면적 그리고 지상부와 지하부의 생체중 및 건물중을 측정하였다. 초장은 지제부에서 식물체의 생장점까지의 높이를 측정하였다. 엽면적은 엽면적 측정기(LI-3000, LICOR Inc., Lincoln, NE, USA)를 이용하여 측정하였다. 경경은 버니어 캘리퍼스(CD-20CPX, Mitutoyo Co., Ltd., Kawasaki, Japan)를 사용하여 측정하였으며, 생체중 및 건물중은 전자저울(EW220-3NM, Kem&Sohn GmbH., Planegg, Germany)을 이용하여 측정하였다. 건물중은 70℃ 항온 건조기(Venticell-222, MMM Medcenter Einrichtungen GmbH., Planegg, Germany)에서 72시간 건조 시킨 후 측정하였다. 광도와 CO2 농도에 따른 묘의 뿌리 발달 정도를 확인하기 위해, 지하부를 스캐너(Expression 12000XL professional scanner, Epson America Inc., Los Alamitos, CA, USA)로 취득한 이미지 데이터를 WinRhizo Pro 2020 뿌리 형태 분석 시스템(Regent Instruments Inc., Sainte-Foy, QC, Canada)을 이용하여 처리 별 총 근장, 근권 표면적, 평균 근경, 근권부 부피, 근단 수를 측정하였다. 묘의 충실도(compactness), T/R율, 엽면적비(leaf area rate, LAR)는 농촌진흥청 농업과학기술 연구조사 분석기준(RDA, 2012)을 기초로 하여 아래의 계산식으로 산출하였다.
3. 실험설계 및 통계분석
실험은 처리당 50개체씩 3반복의 완전임의배치법으로 배치하였으며 처리당 균일한 6개체의 묘를 채취하여 조사하였다. 통계분석은 SAS 프로그램(SAS 9.4, SAS Institute Inc., Cary, NC, USA)을 이용하여 분산분석(ANOVA)을 실시하였고, 평균 간 비교는 Tukey’s test를 이용하여 5% 유의수준에서 각 처리 간 유의성을 검증하였다. 그래프는 SigmaPlot 프로그램(SigmaPlot 14.5, Systat Software Inc., San Jose, CA, USA)을 이용하여 나타냈다.
결과 및 고찰
1. 지상부 생육
처리 22일 차 오이 묘의 초장과 경경은 CO2 농도와 광도 변화에 따른 유의적 차가 나타나지 않았다(Table 2). 엽수는 CO2를 처리하였을 때 광도 300µmol·m-2·s-1 처리구에서 유의적으로 많았으며, 엽면적은 CO2 농도 1,500µmol·mol-1에서 가장 넓었다. 지상부와 지하부의 생체중은 CO2 농도와 광도가 증가함에 따라 증가하는 경향을 보였으며 광도 300µmol·m-2·s-1 처리와 CO2 농도 1,500µmol·mol-1에서 가장 높은 생체중을 나타냈다. 지상부와 지하부의 건물중은 CO2 농도보다 광도의 영향이 더욱 뚜렷하였으며, 광도가 증가함에 따라 증가하는 경향을 보였다. 반면, 토마토 묘의 초장은 광도 300µmol·m-2·s-1 처리에서 가장 짧았다(Table 3). 토마토 묘의 엽수는 CO2 무처리에서 광도가 증가함에 따라 유의적으로 많은 엽수를 나타내었다. 하지만 CO2 농도 1,000과 1,500µmol·mol-1에서는 처리 간에 통계적인 유의성을 확인할 수 없었다. 토마토의 생체중과 건물중은 오이와 유사하게 CO2 농도보다 광도의 영향이 뚜렷하였으며, 광도가 300µmol·m-2·s-1까지 증가함에 따라 생체중과 건물중이 증가하는 경향을 보였다. 토마토 묘의 경경과 엽면적은 처리 간에 유의적인 차이가 없었다. CO2 농도의 증가가 작물의 생육을 증가한다는 결과는 배추, 고추, 무 등의 다른 재배 작물에서도 보고된 바 있다(Oh 등, 2016; Piñero 등, 2014; Lee 등, 2009). 배추의 경우 지상부의 생체중이나 엽면적이 대기 중 CO2 농도가 증가하였을 때 향상되었으며(Oh 등, 2016), 고추와 무에서도 엽장이나 엽면적이 증가하는 등 생장 반응에 긍정적인 효과를 나타낸다고 보고하였다(Piñero 등, 2014; Lee 등, 2009). 이러한 고농도 CO2에서의 생육 증가는 엽면적의 확장과 엽두께가 두껍게 발달하는 등의 형태적 특징을 보인다(Poorter, 1993). 또한 Jang 등(2014)은 오이 접목묘에 조사되는 광도가 증가함에 따라 엽수, 경경, 엽면적, 건물중 등이 증가한다고 보고하였으며, Fan 등(2013)은 토마토 육묘 시 높은 광도가 도장을 억제하고 생체중, 건물중 등의 생육을 증가시킨다고 보고하였다. 그리고 엽수의 경우 생육 단계의 척도가 될 수 있는데(An 등, 2021), 본 연구에서 파종 후 26일 차 오이와 토마토 묘의 엽수는 각각 3-4매엽, 4-6매엽을 나타냈다. 이는 한국 겨울 관행 육묘 시 파종 약 30일 내외에 본엽 3-4매(RDA, 2021), 파종 약 26일 차 토마토 묘의 엽수가 약 3-4매(An 등, 2021)인 것과 비교하여 CPPS에서 육묘 시 생육기간이 단축될 수 있음을 기대할 수 있다. 작물의 광합성은 광 에너지를 이용하여 동화작용을 통해 당을 비롯한 탄수화물을 만드는 과정으로 광, CO2, 수분을 주로 이용하기 때문에 광도와 CO2 농도가 복합적으로 증가함에 따라 광합성량도 증가한다(Makino와 Mae, 1999; Yamori와 Shikanai, 2016). 광합성을 통해 축적된 광합성 산물은 작물의 각 기관에 축적되어 작물의 생육과 대사 작용 촉진에 다양하게 이용된다(Lian과 Tanaka, 1967). 본 실험의 결과 또한 앞선 연구들과 유사하게 CO2 농도와 광도가 증가함에 따라 오이와 토마토의 전반적인 지상부 생육이 향상된 것으로 판단된다.
Table 2.
Growth characteristics of cucumber seedlings as affected by CO2 concentrations and light intensities at 22 days after treatment (n = 6).
CO2 concentration (µmol‧mol-1) |
Light intensity (µmol‧m-2‧s-1) |
Plant height (cm) |
Stem diameter (mm) | No. of leaves |
Leaf area (cm2/plant) | Fresh weight (g) | Dry weight (g) | |||
Shoot | Root | Shoot | Root | |||||||
Ambient | 100 | 3.3 abz | 4.2 | 4.0 a | 122.1 b | 6.72 cd | 0.54 cd | 1.92 bc | 0.067 ab | |
200 | 3.8 ab | 4.4 | 3.3 ab | 135.0 ab | 7.75 b-d | 0.66 b-d | 2.05 bc | 0.065 ab | ||
300 | 3.3 ab | 5.5 | 3.7 ab | 143.6 ab | 8.31 a-c | 0.98 a-c | 3.58 a | 0.112 a | ||
1,000 | 100 | 2.9 b | 4.6 | 3.0 b | 145.9 ab | 6.31 d | 0.49 d | 1.46 c | 0.043 b | |
200 | 3.4 ab | 5.0 | 3.0 b | 128.0 ab | 7.51 b-d | 0.68 b-d | 2.06 ab | 0.078 ab | ||
300 | 3.2 b | 4.9 | 4.0 a | 127.5 ab | 8.54 ab | 1.09 ab | 2.59 ab | 0.084 ab | ||
1,500 | 100 | 3.5 ab | 4.6 | 3.0 b | 159.2 a | 7.49 b-d | 0.55 cd | 1.53 c | 0.043 b | |
200 | 3.5 ab | 4.8 | 3.0 b | 151.1 ab | 9.12 ab | 0.87 a-d | 1.96 bc | 0.086 ab | ||
300 | 4.3 a | 4.7 | 4.0 a | 159.1 a | 11.01 a | 1.28 a | 2.31 ab | 0.090 ab | ||
F-test | CO2 concentration (A) | * | NS | * | *** | *** | ** | NS | NS | |
Light intensity (B) | NS | NS | *** | NS | *** | *** | *** | *** | ||
A×B | NS | NS | ** | NS | NS | *** | NS | NS |
Table 3.
Growth characteristics of tomato seedlings as affected by CO2 concentrations and light intensities at 22 days after treatment (n = 6).
CO2 concentration (µmol‧mol-1) |
Light intensity (µmol‧m-2‧s-1) |
Plant height (cm) |
Stem diameter (mm) | No. of leaves |
Leaf area (cm2/plant) | Fresh weight (g) | Dry weight (g) | |||
Shoot | Root | Shoot | Root | |||||||
Ambient | 100 | 14.1 acz | 4.00 | 3.7 c | 119.23 | 4.60 c | 0.46 c | 0.60 c | 0.04 c | |
200 | 16.0 ab | 4.79 | 5.3 ab | 134.63 | 7.29 a-c | 1.00 ab | 1.06 a-c | 0.09 a | ||
300 | 12.5 c | 3.99 | 5.7 a | 179.53 | 9.71 a | 1.05 a | 1.29 a-c | 0.09 a | ||
1,000 | 100 | 16.6 a | 4.31 | 4.0 bc | 123.84 | 5.70 bc | 0.55 bc | 0.61 c | 0.04 c | |
200 | 16.0 ab | 4.20 | 3.7 c | 124.05 | 6.37 a-c | 0.73 a-c | 0.80 a-c | 0.05 ab | ||
300 | 14.2 a-c | 4.27 | 4.3 a-c | 158.84 | 8.33 ab | 1.00 ab | 1.43 ab | 0.10 ab | ||
1,500 | 100 | 14.2 a-c | 4.14 | 4.3 a-c | 148.45 | 6.52 a-c | 0.62 a-c | 0.64 bc | 0.04 bc | |
200 | 14.0 a-c | 4.62 | 5.0 a-c | 135.89 | 8.19 a-c | 0.92 a-c | 0.99 a-c | 0.07 a-c | ||
300 | 13.2 bc | 4.80 | 4.0 bc | 164.83 | 8.20 a-c | 1.00 ab | 1.52 a | 0.11 a | ||
F-test | CO2 concentration (A) | * | NS | * | NS | * | * | ** | ** | |
Light intensity (B) | ** | NS | NS | NS | ** | *** | *** | *** | ||
A×B | NS | NS | ** | NS | NS | NS | NS | NS |
2. 지하부 생육
Fig. 3과 Fig. 4는 CO2 농도와 광도 처리 22일 후의 오이와 토마토 묘의 뿌리를 스캔하여 나타낸 이미지이다. 묘소질은 잎 크기, 줄기 길이 등과 더불어 뿌리의 발육 상태를 기준으로 판단할 수 있고(RDA, 2020), 너무 어린 묘의 경우 정식 후 뿌리의 활력과 양수분 흡수가 지나쳐 잎과 줄기가 웃자라는 문제가 있다(RDA, 2021). 따라서 묘를 이용하는 대부분의 농가에서 육안으로 묘 뿌리를 확인하는 것은 매우 중요하다. 오이 묘의 평균 근경을 제외한 총 근장, 근권 표면적, 근권부 부피, 그리고 근단 수와 같은 뿌리 발달은 CO2 무처리와 광도 300µmol·m-2·s-1 처리에서 가장 높았으며(Fig. 5), 근권 표면적과 근권부 부피의 경우 100µmol·m-2·s-1 처리에서 낮은 경향을 보였다(Fig. 5B와 5D). 토마토 묘 또한 광도가 300µmol·m-2·s-1까지 증가함에 따라 평균 근경을 제외한 총 근장, 근권 표면적, 근권부 부피, 그리고 근단 수와 같은 뿌리 발달이 모두 증가하는 경향을 보였으며(Fig. 6), 총 근장, 근권 표면적, 그리고 근권부 부피의 경우 CO2 1,500µmol·mol-1와 광도 300µmol·m-2·s-1 처리에서 가장 높았다(Fig. 6A, 6B와 6D). 증가한 광은 잎을 통하여 흡수되고 줄기와 뿌리의 관다발을 통해 지하의 뿌리까지 전달되어 뿌리에 존재하는 피토크롬 B 광수용체가 HY5 전사인자를 활성화하고, 일련의 유전자 발현을 촉진함으로써 뿌리 생장과 발달을 조절하여 식물의 생장을 촉진 시키고 뿌리를 발달시켰을 것으로 판단된다(Lee 등, 2016a). 총 근장, 근권 표면적, 근권부 부피, 그리고 근단 수와 같은 뿌리 발달은 무기이온의 흡수를 촉진하며, 스트레스 저항, 그리고 토양 미생물과의 상호작용 등에 영향을 주어 정식 후 뿌리 활착과 양분 흡수에 긍정적인 효과를 제공할 수 있다(Atwater 등, 2015; Busso 등, 2001; Choi와 Cho, 2019; Foxx와 Kramer, 2020; Hetrick, 1991). 하지만 오이의 경우 CO2 1,500µmol·mol-1처리에서는 광도 증가에 따른 총 근장과 근단 수의 증가 효과를 관찰할 수 없었다(Fig. 5A와 5E). 이는 CO2 시비가 작물생육 속도를 37% 증가시킨다는 가장 일반적인 효과(Poorter, 1993)와 더불어 재배기간이 비교적 짧은 과채류인 오이의 특성(RDA, 2021)이 영향을 주었을 것으로 판단된다. 따라서 CO2 농도와 광도 조절 시 오이 묘의 초기 생육과 뿌리 발달 변화를 관찰하는 추가 실험이 필요할 것으로 판단된다. CO2 농도의 경우 오이와 토마토 묘의 뿌리 발달에 유의적인 효과는 관찰할 수 없었다(Fig. 5와 6). 오이 묘의 CO2와 광포화점은 각각 1,200-1,300µmol·mol-1, 665µmol·m-2·s-1로 보고되고(Lee와 Kim, 2012; Lim 등, 2000), 토마토 묘의 CO2와 광포화점은 각각 1,200-1,500µmol·mol-1, 847µmol·m-2·s-1로 보고되고 있다(Lee와 Kim, 2012; Ting 등, 2017; Wang 등, 2013). 본 연구에서 CO2 농도는 오이와 토마토 두 작물의 CO2 포화점 근처(1,000, 1,500µmol·mol-1)로 공급했지만, 광도는 최대 300µmol·m-2·s-1로 광포화점보다 낮았기 때문에 식물의 생장은 필요 요소 중 다른 모든 요소가 충족되었더라도 가장 부족한 요소에 의해 결정된다는 리비히의 최소율의 법칙에 의거하여(Salisbury, 1988), CO2 농도의 증가보다 광도의 증감에 영향을 받은 것으로 판단된다. 이러한 결과는 뿌리로의 동화산물 이동이 CO2 농도의 증가가 광도의 증가만큼 효과적이지 않았다는 Poorter와 Nagel(2000)의 연구 결과와도 유사하였다. 따라서 오이와 토마토 묘 단계에서의 뿌리는 CO2 농도보다 광도의 영향을 받았으며, 광도 증가에 따라 총 근장, 근권 표면적, 근권부 부피, 그리고 근단 수가 발달하는 경향을 나타냄을 확인하였다.
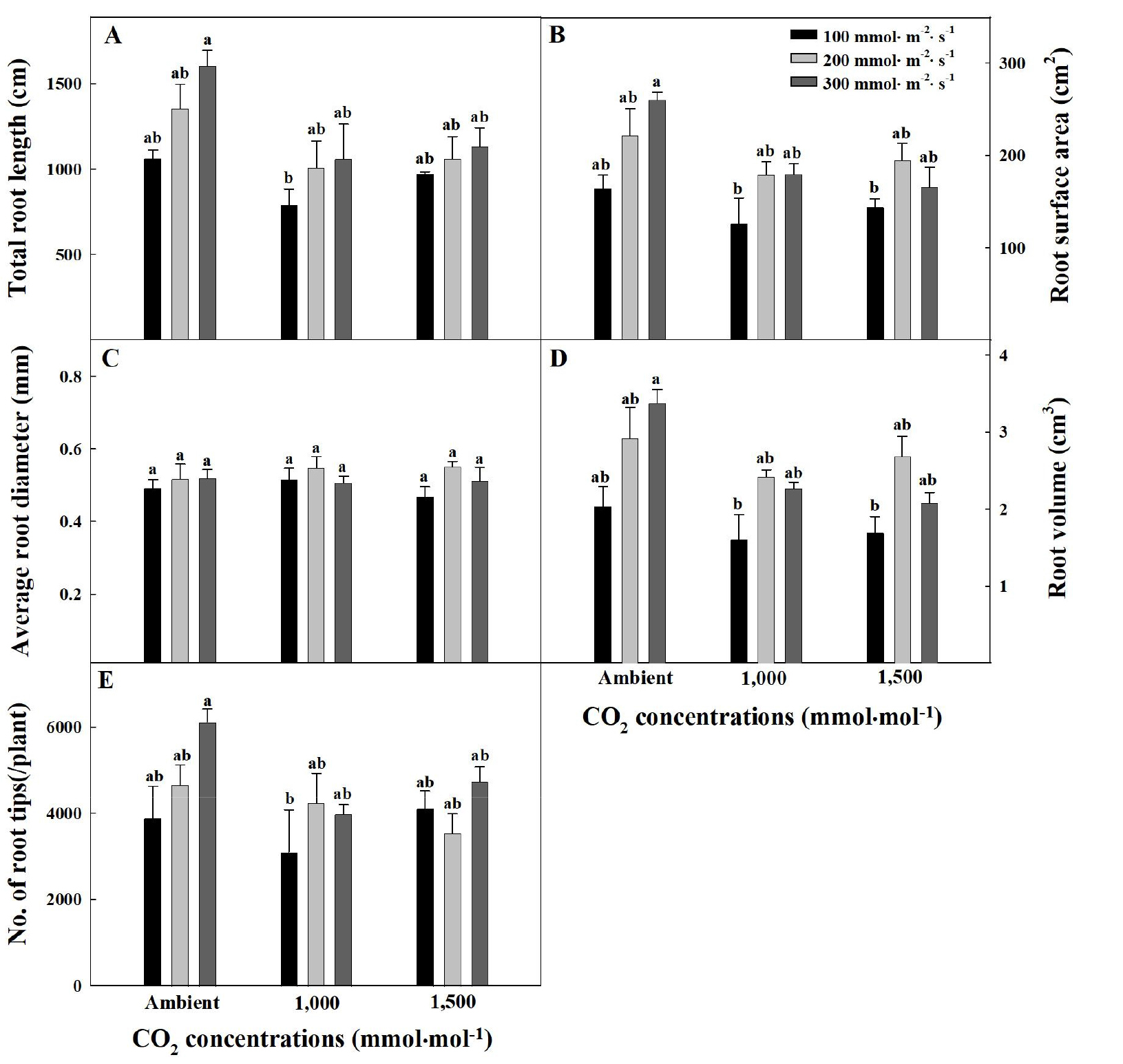
Fig. 5.
Total root length (A), root surface area (B), average root diameter (C), root volume (D), and number of root tips (E) of cucumber seedlings under different CO2 concentrations and light intensities at 22 days after treatment. Vertical bars represent the stand deviation of the mean (n = 6). Different letters above bars indicate significant differences by the Tukey’s test at p ≤ 0.05.
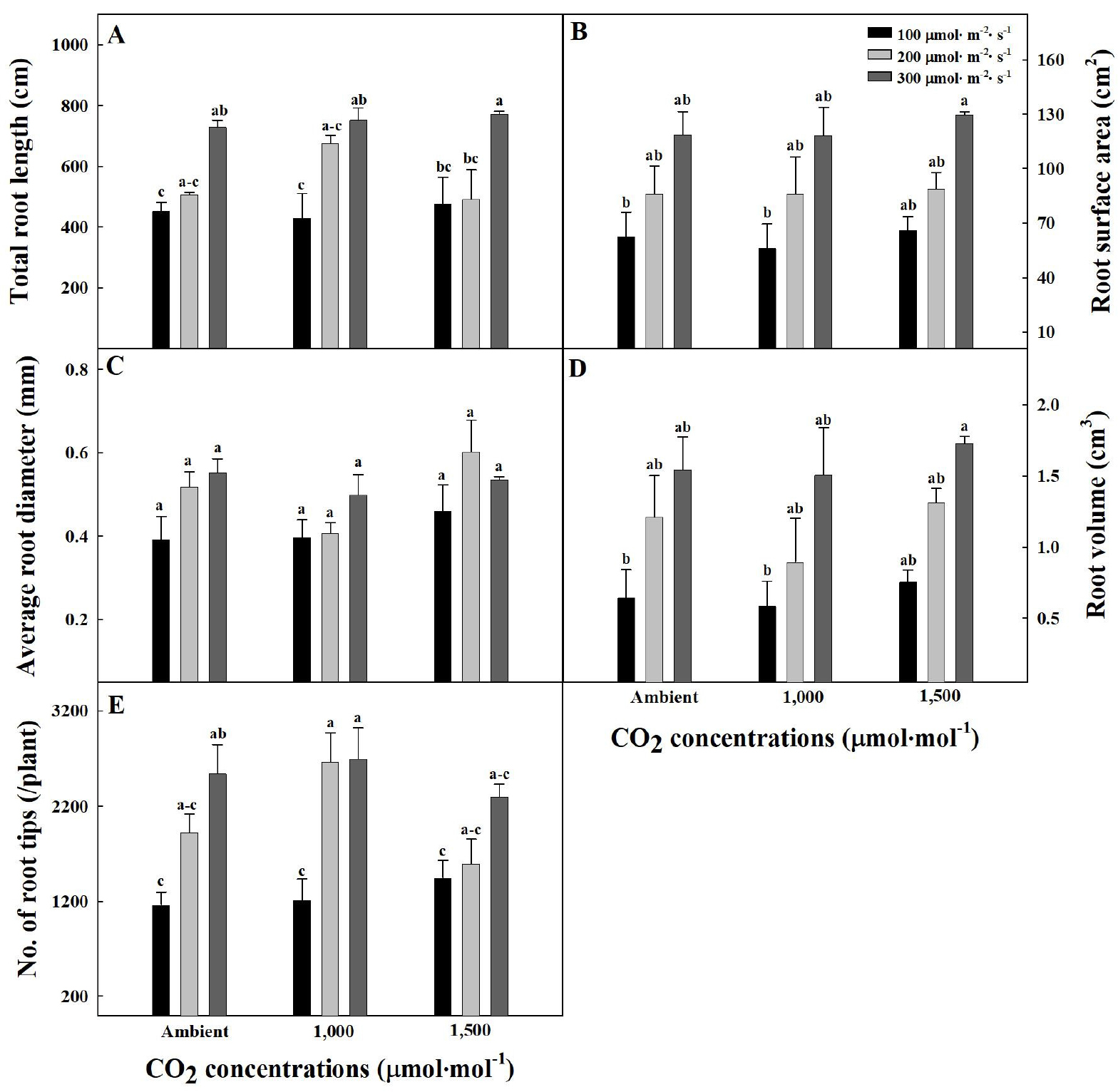
Fig. 6.
Total root length (A), root surface area (B), average root diameter (C), root volume (D), and number of root tips (E) of tomato seedlings under different CO2 concentrations and light intensities at 22 days after treatment. Vertical bars represent the stand deviation of the mean (n = 6). Different letters above bars indicate significant differences by the Tukey’s test at p ≤ 0.05.
3. 묘소질
CO2 농도와 광도 처리 22일 후의 오이와 토마토 묘의 충실도는 오이와 토마토 묘 모두 광도가 증가함에 따라 증가하는 경향이 나타났다(Fig. 7A와 7B). 하지만 T/R율은 오이와 토마토 묘 모두 CO2 농도와 광도에 따른 유의적인 차이가 없었다(Fig. 7C와 7D). LAR은 오이와 토마토 묘 모두 광도가 증가함에 따라 감소하는 경향이 나타났다(Fig. 7E와 7F). 오이와 토마토 묘의 충실도, T/R율, 그리고 LAR 모두 CO2 농도보다 광도의 영향이 뚜렷하였으며, 300µmol·m-2·s-1까지의 광도 증가는 묘소질을 향상시켰다. 충실도, T/R율, 그리고 LAR는 묘소질을 확인하는 지표로 이용되며, 충실도가 높을수록 키가 작고 줄기가 두꺼운 고품질 묘임을 나타낸다(Lee 등, 2016b; Zhang 등, 2003). LAR은 엽면적을 전체 건물중으로 나눈 것으로 값이 낮을수록 잎의 두께가 두꺼운 고품질 묘이다(Jo 등, 2021). 본 실험에서 광도가 증가함에 따라 묘소질이 향상되었는데, 이는 광도가 증가함에 따라 광합성 촉진 및 도장이 억제된다는 Steinger 등(2003)의 보고와 유사하였다. 또한 광도의 증가는 잎의 책상 조직과 해면조직을 발달시켜 잎을 두껍게 하는 등 잎 세포의 배열과 식물의 형태에도 영향을 미치기 때문에(Lee 등, 1985; Li 등, 2009). 광도 300µmol·m-2·s-1 처리에서 낮은 LAR을 나타낸 것으로 판단된다. 반면, CO2 농도의 증가는 오이와 토마토의 묘소질에 큰 영향을 주지 않았다. Son과 Kim(1998)은 광합성이 온도, CO2 농도, 광, 습도 등 다양한 환경의 영향을 받는데, 그중 광도가 광합성에 가장 큰 영향을 미치는 요인이라고 보고하였다. 따라서 본 연구에서도 CPPS내 CO2 농도와 광도의 증가가 오이와 토마토의 엽수, 엽면적, 그리고 생체중과 같은 전반적인 생육을 증가시켰지만, 300µmol·m-2·s-1까지의 광도 증가가 CO2 농도보다 충실도와 LAR과 같은 묘소질 향상에 큰 영향을 준 것을 확인하였다.
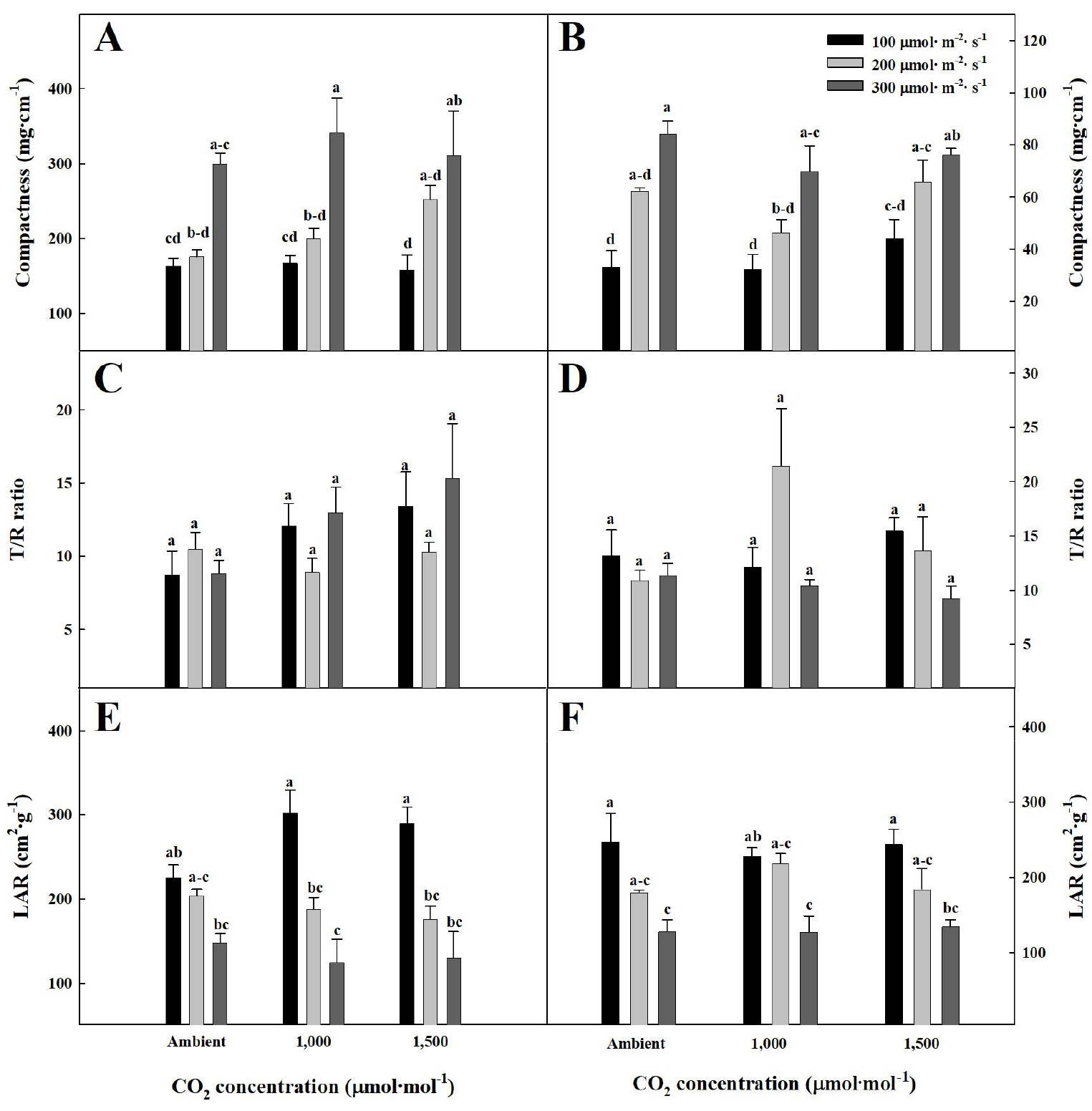
Fig. 7.
Compactness (A), T/R ratio (C), and LAR (leaf area rate) (E) of cucumber seedlings, and compactness (B), T/R ratio (D), and LAR (F) of tomato seedlings under different CO2 concentrations and light intensities at 22 days after treatment. Vertical bars represent the stand deviation of the mean (n = 6). Different letters above bars indicate significant differences by the Tukey’s test at p ≤ 0.05.
결론적으로 CPPS내에서 오이와 토마토 묘의 생육, 묘소질 그리고 지하부 발달은 CO2 농도보다 광도의 영향을 크게 받는 것으로 판단되며, 광도가 증가함에 따라 생육, 묘소질, 그리고 뿌리 발달이 증가하는 경향을 나타냈다. 이러한 결과는 지상부 생육뿐만 아니라 지하부 발달도 고려한 CPPS 묘 생산 연구의 보충 자료가 될 수 있으며, CO2 농도보다는 광도 조절을 통하여 좀 더 경제적으로 고품질 묘를 생산할 수 있을 것으로 예상된다. 최근 식물공장 상품 개발자 측면이나 육묘업체의 새로운 생산 방법 연구 등 다양한 방면에서 CPPS를 활용한 묘 생산 연구는 지속적으로 진행되고 있다. 따라서 본 연구의 CPPS내 CO2와 광도에 따른 오이와 토마토 묘의 생육 결과는 기존 묘소질을 하락시키지 않고 생산 속도를 앞당기며, 뿌리 발달도 고려한 경제적인 고품질 묘 생산 연구에 기초자료가 될 수 있다.