Introduction
Materials and Methods
1. Plant Materials and Experimental Design
2. Measurement of Plant Growth
3. Measurement of Fruit Productivity
4. Measurement of Fruit Quality
5. Statistical analysis
Results
1. Vegetative Growth of Plants as Affected by Propagation Method and Cultivation System
2. Fruit Productivity
3. Fruit External Quality
4. Fruit Internal Quality
Discussion
Conclusions
Introduction
Strawberry (Fragaria × ananassa Duch.) is one of the major fruit crops in Korea. It is a good crop with high economic returns from both domestic and exported markets. In 2018, domestic production value of strawberry was about 1.3 trillion KRW (official currency of the Republic of Korea) in Korea (KOSIS, 2018).
In Korea, the traditional production of strawberry transplants is conducted from the end of March to the beginning of September. This traditional method (pinning propagation) requires transplantation of the mother plants, anchoring of runner plants, and separation of runner plants from the mother plants. These processes require more than six months (Na et al., 2014; Park and Choi 2015). However, cutting propagation method is known to require only three to four months to complete those steps described above (Kim et al., 2018). For cutting propagation, runner plants are harvested from mother plants and stuck in substrate. After keeping them in a fogged tunnel for 9-12 days, they will be well-rooted (Wei et al., 2020). Then, they can be cultivated until transplantation. Recently, study was also focused on the improvement of root development during cutting propagation of strawberry, which suggested that hydrogen sulfide could promote the root development (Hu et al., 2020). Although cutting propagation consumes less time and labor, the growth, fruit productivity, and fruit quality of strawberry as affected by these two propagation methods have not been investigated.
Cultivation system plays an important role in influencing quality and yield of fruit. Soil cultivation is the traditional way to cultivate strawberry, which takes the advantages of low investment and short-term economic benefit. However, studies have revealed many disadvantages of soil cultivation system such as pathogen problems (Pastrana et al., 2016) and the high temperature caused by mulching film (Muñoz et al., 2017). The hydroponic system with substrate can be used to cultivate crops without soil, which is currently in practice all over the world (Rouphael et al., 2004; Rouphael et al., 2006). It can also be defined as a kind of cultivation method that meets mineral requirements of specific crops. The nutrient solution of hydroponic system is recyclable, which is economical and environmentally friendly (Zekki et al., 1996). It was reported that hydroponic cultivation can increase productivity and improve fruit quality through intensive and effective crop managements (Domis et at., 2002). The hydroponic cultivation system requires higher investment but has the advantages of shorter growth period, higher yields per unit area, and better fruit quality achieved by managing nutrients suitable for specific crops with potentially less soil diseases and subsequent crop damages (Schwarz, 1995; Lee et al., 2011). Generally, hydroponic cultivation can produce yields 2 to 3 times yields more than soil cultivation system in Korea. The strawberry plants can also be cultivated at an elevated height, which benefits growers by easy cultivation and picking and less fatiguing (Yoon et al., 2004; Lee et al., 2010). Moreover, hydroponic cultivation system makes better use of space and location and saves use of water, insecticide, and herbicides (Benke and Tomkins, 2017). Therefore, it is interesting to investigate the growth, fruit productivity, and fruit quality of strawberry as affected by soil cultivation and hydroponic cultivation systems.
This study was conducted using one of the most widely cultivated strawberry cultivar ‘Seolhyang’ in Korea. The runner plants were propagated by cutting propagation and pinning propagation. Then, they were transplanted into soil and hydroponic cultivation systems. Plant growth and fruit performance were measured during the whole harvest period. The results of this study can provide useful guidance for commercial production of strawberry.
Materials and Methods
1. Plant Materials and Experimental Design
The experiment was conducted in Jinju, Korea (35°09′32′′N, 127°56′49′′E) by using strawberry ‘Seolhyang’. To investigate the performance of strawberry as affected by propagation method and growing medium during the whole harvest period. The cutting-propagated and pinning- propagated transplants were respectively transplanted into soil and hydroponic cultivation systems. There were three different positions in each system which were used as three replicates (40 plants in each replicate). Data collected from each replicate were used for statistical analysis. These runner plants were obtained from a commercial strawberry grower in Jinju. The schedule of this experiment was shown in Fig. 1. For soil cultivation system, strawberry plants were planted in the field under a high tunnel. The hydroponic cultivation system installed in a high tunnel and used BVB Medium (Bas Van Buuren Substrates, EN-12580, De Lier, The Netherlands) as the substrate. The temperature ranged from 7 (nighttime) to 40 ℃ (daytime), the relative humidity ranged from 82.2 (daytime) to 94.2 % (nighttime), and the average light intensity ranged from 212.2 to 329.9 μmol·m−2·s−1 PPFD during the whole cultivation period. The composition of the nutrient solution was as follows (in mg‧L-1): 599.00 NH4NO3(Ca(NO3)2‧2H2O)5‧, 258.00 KNO3, 36.00 NH4NO3, 23.08 Fe-EDTA, 228.00 K2SO4, 107 KH2PO4, 330.00 Mg(NO3) 6H2O, 2.94 H3BO3, 2.00 MnSO4‧4H2O, 0.87 ZnSO4‧7H2O, 0.16 CuSO4‧5H2O, 0.10 Na2MoO4‧2H2O. At the beginning of transplantation, the EC was 0.6 dS‧m-1 with an increment of 0.1 dS‧m-1 per week until it reached 1.4 dS‧m-1. The pH of nutrient solution changed along with the EC, it ranged from 5.8 to 6.1. The irrigation device (SH-1700, Shin Han A-Tec, Changwon, Korea) was used for irrigation. The frequency of irrigation changed along with the temperature, it ranged from three times to eight times a day, each time lasted for 2.5 or 3.0 minutes. The drip tape with 15-cm distance of drip holes was used, each hole could give plants 23 mL of nutrient solution per minute.
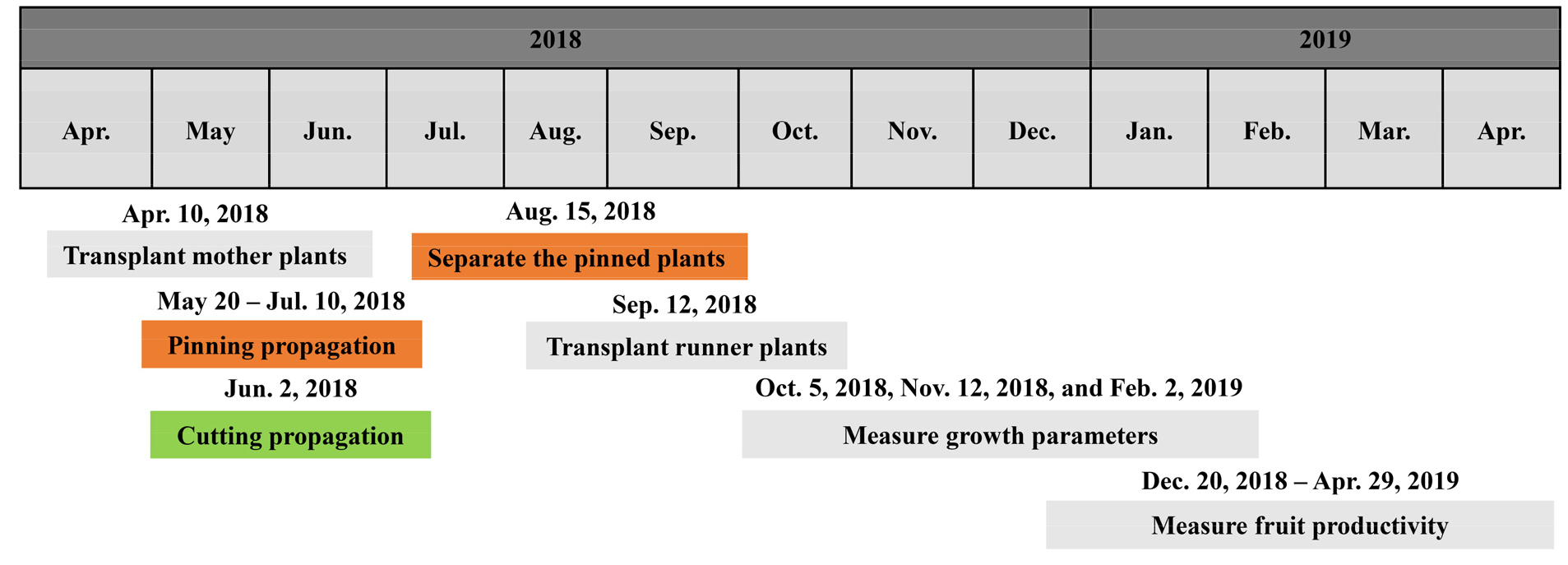
Fig. 1.
Schedule of this experiment. Pining propagation, cutting propagation, and cultivation of mother plants and runner plants were conducted in a propagation greenhouse. The propagated runner plants were respectively transplanted into soil and hydroponic cultivation systems on September 12, 2018. Pinning propagation: Pinning the runner plants in the substrate. Cutting propagation: Removing runner plants from mother plants and sticking them in the substrate.
2. Measurement of Plant Growth
Growth parameters such as plant height, leaf length, leaf width, leaf chlorophyll content, and crown diameter were measured on October 5 and on November 12, 2018 (1 and 2 months after transplantation, respectively). Also, they were measured at the middle of the harvest period on February 2, 2019. The measurements were finished before noon during the day. The full-expanded leaves were used to measure the leaf growth characteristics including leaf length, leaf width, chlorophyll content (SPAD), and number of leaves. Crown diameter was measured by digital Vernier calipers (CD- 20CPX, Mitutoyo Korea Corp., Gunpo, Korea). Leaf chlorophyll content was measured by the SPAD instrument (SPAD-502, Konica Minolta Inc., Tokyo, Japan). Total of 15 plants from three replicates per treatment were measured.
3. Measurement of Fruit Productivity
The fruit harvest started on December 20, 2018 and continued at four to five days of interval until April 29, 2019. The harvest was finished before noon, and number of fruits, total fruit weight, and unmarketable fruit weight were counted and measured on the same day. The fruit weight was measured by a digital balance (ENTRIS224i-1S, Sartorius Lab Instruments GmbH and Co., KG, Goettingen, Germany). Unmarketable fruits were divided into 3 groups including decayed, malformed, and small fruits. The small fruits were those with the weight less than 10 grams. All fruits were harvested from 120 plants in three replicates per treatment.
4. Measurement of Fruit Quality
The fruit quality was measured at one month of interval from January to April of 2019. Fruit length and diameter were measured by digital Vernier calipers. Fruit firmness was measured by a firmness meter (RHEO TEX SD-700, Sun Scientific Co., Ltd., Tokyo, Japan) with 3 mm probe. Fruit firmness was calculated and indicated by the N value. To measure the soluble solid content (SSC) and acidity, five fresh fruit samples were collected from each treatment and wrapped in a cheese cloth. The fruit juice was collected by pressing the wrapped fruits. Then, the juice was measured by a hand refractometer (PR-201α, Atago Co., Ltd., Tokyo, Japan) for SSC and a hand fruit acidity meter (GMK-835N, G-WON Hitech Co., Ltd., Seoul, Korea) for the acidity. The SSC was indicated by °Brix. Color indexes of the fruit skin including lightness, a* (red to green index), b* (yellow to blue index), H (hue angle between a* and b*), and C* (chroma index) were measured by a colorimeter (CR-410, Konica Minolta, Inc., Tokyo, Japan). Hue angle and chroma index was calculated by the following formulas:
H = tan-1(b*/a*) (Carreño et al., 1995)
C* = (a*2 + b*2)1/2 (Carreño et al., 1995)
The measurements were conducted right after the harvest.
5. Statistical analysis
A statistical analysis was conducted using the SAS program (SAS 9.4, SAS Institute Inc., Cary, NC, USA). Data were analyzed by an analysis of variance (ANOVA). The main factors of plant growth and fruit productivity were analyzed by the Duncan’s multiple range test at P ≤ 0.05. Differences of fruit quality as affected by cultivation system were checked by the Student′s t-test at P ≤ 0.05.
Results
1. Vegetative Growth of Plants as Affected by Propagation Method and Cultivation System
Vegetative growth parameters of strawberry plants were shown in Table 1. The F-test showed that cultivation system had significant effects on all the tested parameters two months after transplantation (P ≤ 0.05).
Table 1.
Vegetative growth of strawberry as affected by propagation method and cultivation system on October 5, 2018. Data were represented by the mean ± standard error (n=15). Comparison of means was conducted only when significant difference was found in each factor or interaction. The capital letters followed by means represent differences in propagation method. The ns and * followed by means represent non-significant and significant difference in cultivation system. The lowercases followed by means represent differences in propagation method and cultivation system.
zNS, *, **, and *** represent non-significant or significant at P ≤ 0.05, 0.01, 0.001, respectively. The analyses were performed by the ANOVA
analysis, and verified by the Duncan’s multiple range test at P ≤ 0.05.
Table 2.
Vegetative growth of strawberry as affected by propagation method and cultivation system on November 12, 2018. Data were represented by the mean ± standard error (n=15). Comparison of means was conducted only when significant difference was found in each factor or interaction. The capital letters followed by means represent differences in propagation method. The ns and * followed by means represent non-significant and significant difference in cultivation system. The lowercases followed by means represent differences in propagation method and cultivation system.
zNS, *, **, and *** represent non-significant or significant at P ≤ 0.05, 0.01, 0.001, respectively. The analyses were performed by the ANOVA
analysis, and verified by the Duncan’s multiple range test at P ≤ 0.05.
Table 3.
Vegetative growth of strawberry as affected by propagation method and cultivation system on February 2, 2019. Data were represented by the mean ± standard error (n=15). Comparison of means was conducted only when significant difference was found in each factor or interaction. The capital letters followed by means represent differences in propagation method. The ns and * followed by means represent non-significant and significant difference in cultivation system. The lowercases followed by means represent differences in propagation method and cultivation system.
zNS, *, **, and *** represent non-significant or significant at P ≤ 0.05, 0.01, 0.001, respectively. The analyses were performed by the ANOVA
analysis, and verified by the Duncan’s multiple range test at P ≤ 0.05.
The plant height of cutting-propagated transplants was higher (16.6%) in soil cultivation system as compared with that in hydroponic cultivation system on October 5, 2018, while the pinning-propagated plants were higher (16.6%) in hydroponic cultivation system as compared with that in soil cultivation system on November 12, 2018. The crown diameter of cutting-propagated plants was higher (24.0%) as compared with pinning-propagated plants in soil cultivation system on October 5, 2018, while it was significantly greater (cutting propagation: 31.0%, pinning propagation: 15.0%) in hydroponic cultivation system as compared with that in soil cultivation system on November 12, 2018 (P ≤ 0.05). Leaf length (cutting propagation: 22.5%, pinning propagation: 21.0%) and leaf width (cutting propagation: 16.7%, pinning propagation: 19.8%) were greater in soil-cultivated and hydroponic-cultivated plants on October 5, 2018, regardless of the propagation method, while they were greater in hydroponic cultivation system on November 12, 2018 (leaf length: 12.8% and 16.5%; leaf width: 13.3% and 11.2%), regardless of the propagation method. The pinning- propagated plants had higher leaf length (18.0%) and leaf width (22.2%) in hydroponic cultivation system as compared with that in soil cultivation system on February 2, 2019. Chlorophyll content (SPAD) was increased in hydroponic cultivation system on October 5, 2018 (cutting propagation: 5.5%, pinning propagation: 6.3%), November 12, 2018 (cutting propagation: 4.9%, pinning propagation: 6.8%), and February 2, 2019 (cutting propagation: 11.3%, pinning propagation: 12.6%), regardless of the propagation method. Number of leaves was only increased (cutting propagation: 20.2%, pinning propagation: 101.3%) in hydroponic cultivation system on February 2, 2019, regardless of the propagation method.
2. Fruit Productivity
Total and average fruit weights showed no significant differences in the two propagation methods (P ≥ 0.05), while they were significantly affected by timing and cultivation system (P ≤ 0.05). Strawberry produced by hydroponic cultivation system had a significantly greater total yield per plant (cutting propagation: 38.9%, pinning propagation: 82.0%) than that in soil cultivation system (P ≤ 0.05). The greatest total yield per plant was 516.0 grams in the pinning-propagated plants cultivated in hydroponic system, whereas the lowest total yield per plant was 283.5 grams in the pinning-propagated plants grown in the soil (Fig. 2). Total fruit yield was greatest in March and lowest in December. There were no statistically significant differences in propagation methods except in January. The cutting- propagated plants in soil cultivation system produced more fruits as compared with pinning-propagated plants in January (72.2%) and February (31.8%).
Average fruit weight per fruit showed the same changes as total fruit weight (Fig. 2). Moreover, it decreased rapidly before February, and then decreased gradually until the end of harvest period. There were no statistically significant differences in propagation methods except in December, which showed the pinning-propagated strawberry had higher average fruit weight in hydroponic cultivation system (P ≤ 0.05). It was always higher in hydroponic cultivation as compared with that in soil cultivation system. The greatest differences were found before February.
Cultivation system was the main factor that affected amount of unmarketable fruits. Propagation method showed limited effects on unmarketable fruits except that pinning- propagated strawberry had less malformed fruits (P ≤ 0.05). Total unmarketable fruit ratio was significantly greater in soil cultivation system (cutting propagation: 279.0%, pinning propagation: 320.0%) than that in hydroponic cultivation system (P ≤ 0.05, Fig. 3). Small fruits were the primary unmarketable fruits in soil cultivation system, while malformed fruits were the primary unmarketable fruits in hydroponic cultivation system.
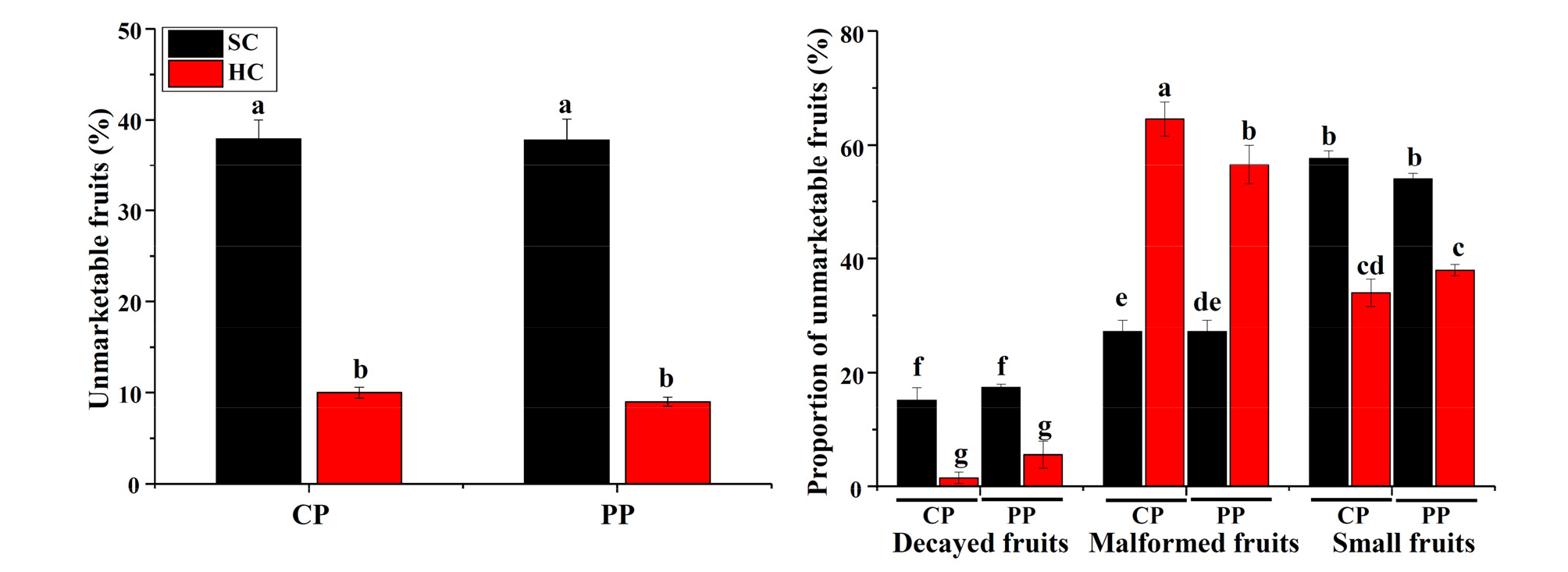
Fig. 3.
Unmarketable fruit ratio (%) and proportion of unmarketable fruits as affected by propagation method and cultivation system. SC, soil cultivation; HC, hydroponic cultivation; CP, cutting propagation; PP, pinning propagation. Different letters represent significant difference according to the Duncan’s multiple range test at P ≤ 0.05.
3. Fruit External Quality
Fruit external quality showed no significant differences in the two propagation methods (P ≥ 0.05), while it was significantly affected by timing and cultivation system (P ≤ 0.05, Fig. 4). The fruit length and fruit diameter were not statistically different between cutting propagation and pinning propagation method (P ≤ 0.05), they were greater in the hydroponic cultivation system than that in soil cultivation system. The fruit firmness was the greatest on February 27, 2019 and gradually decreased as the harvest period progressed, it was generally greater in soil cultivation system than that in hydroponic cultivation system (P ≤ 0.05).
The color indexes of fruit skin showed statistically different in the two cultivation systems. The lightness (L*) was only statistically different between cutting propagation and pinning propagation method on January 22, 2019. Fruits harvested in hydroponic cultivation system showed greater a* index than that in soil cultivation system. Consistently, lower H (change in color from green to red) was found in hydroponic cultivation system on February 27, 2019. Moreover, b* and C* were found to be higher in hydroponic cultivation system on February 27, 2019. These results indicated that the fruits produced by hydroponic cultivation system were more red in color.
4. Fruit Internal Quality
Fruit internal quality showed no significant differences in the two propagation methods (P ≥ 0.05), while it was significantly affected by timing and cultivation system (P ≤ 0.05, Fig. 5). In general, SSC decreased and acidity increased as time goes on. Pinning-propagated strawberry had higher SSC in hydroponic cultivation system as compared to soil cultivation system on January 22 and April 29 (P ≤ 0.05). Cutting-propagated strawberry had higher acidity in soil cultivation system as compared to hydroponic system on January 22, 2019 (P ≤ 0.05).
Discussion
Propagation of transplants is essential for fruit yield of strawberry. Well-propagated transplants have the advantages of early fruiting and producing high yield and high quality of fruits (Hochmuth et al., 2006; Giménez et al., 2009). Our results suggested that the cutting-propagated transplants had better performance on crown diameter, leaf length, and leaf width one month after transplantation, which might result in higher productivity in January.
According to the previous study, there was only a little difference in nutrient quality between the hydroponic and soil cultivation systems (Hochmuth et al., 2006). However, soil cultivation system had increased weed issues compared to the hydroponic cultivation system (Treftz and Omaye, 2015), which might cause nutritional problems for strawberry. The hydroponic cultivation system uses a nutrient solution or soilless substrate as the growing medium to manage weeds which interferes with strawberry growth and eliminates soil-borne pathogen problems (LaMondia et al., 2002). In the hydroponic cultivation system, photosynthesis per unit area and stomatal conductance usually are greater than that in the soil cultivation system (Anver et al., 2005). Previous study on the comparison of two cultivation systems for strawberry reported that the hydroponically cultivated strawberries had a 17% greater yield as compared with the soil-grown strawberries (Treftz and Omaye, 2015). Lee et al. (2011) found that densely planted crops have advantages because the total yield per unit area increased significantly in hydroponic cultivation system. It was also reported that total yield per plant was greater in soil cultivation system, but total yield per unit area was greater in hydroponic cultivation system (Giménez et al., 2009; Lee et al., 2011). However, our results showed that both total yield per plant and average fruit weight were greater in hydroponic cultivation system. In addition, the average fruit weight was dramatically higher in hydroponic cultivation system before February when the price of strawberry was highest during the harvest season. These results indicated that growers can benefit from both yield and price of strawberry fruits by hydroponic cultivation system.
The environmental factors impacted unmarketable fruit number. Strawberry fruits exposed to high relative humidity often suffer from several diseases such as grey mold (Botrytis cinerea), stem-end rot (Gnomoniopsis fructicola), powdery mildew (Podospharea aphanis), and black spot (Colletotrichum acutatum). Strawberry plants also produce fewer and smaller fruits under high temperature condition (Taghavi et al., 2016). It was reported that up to 50% of the ripe fruits can be affected by these diseases (Menzel et al., 2016a; Menzel et al., 2016b). In hydroponic cultivation system, strawberry plants were lifted above the ground and the fruits were hanged in the air, while the fruits of soil-cultivated strawberry were laid on the black mulching film. Thus, fruits cultivated in soil cultivation system faced with higher temperature, which might be the reason for high proportion of small fruits.
Fruit quality is greatly affected by the environmental factors including climatic condition, nutrient, moisture, temperature, cultivation system, and management technique (Wang and Camp, 2000). It has been shown that strawberry cultivated in highland had different characteristics of growth and development as compared with that in plain area (Lee et al., 2005). Our results showed that the plants cultivated in hydroponic cultivation system had higher fruit quality as compared with that in soil cultivation system, which might be caused by the better environmental factors in hydroponic cultivation system. Fruit external and internal quality changed greatly during the harvest period. The overall high quality of fruits was found on February 27, 2019, which suggested that fruit quality of strawberry differed during harvest season. These results were also confirmed by other studies (Wold and Opstad, 2012; Vicente et al., 2014).
Moreover, in this study, the soil and hydroponic cultivation systems were located in two different high tunnels. There were some limitations in comparing these two cultivation systems. The most reliable way of comparison was to place both systems under optimal growing conditions. Therefore, some changes might also be caused by the different conditions of high tunnels. We suggested that future research should be conducted as a trial at a larger level using a split plot design and several tunnels to reduce tunnel influence.
Conclusions
The results of this study showed that the labor-saving propagation method, cutting propagation, had higher productivity of strawberry plants in January, and hydroponic cultivation system produced high-yield and high-quality fruits. Although the initial investment of soil cultivation system is lower, the hydroponic cultivation system has the potential to obtain a better long-term economic benefits.